Demystifying Complex Theories for Students: Electromagnetic Induction
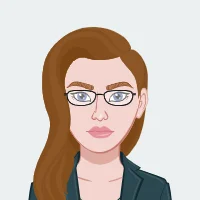
In the intricate realm of physics, the exploration of electromagnetic induction stands as a captivating endeavor, particularly for students navigating the complexities of scientific theories. This fascinating subject revolves around the fundamental principles encapsulated in Faraday's law and Lenz's law, where a dynamic interplay of magnetic fields and electrical currents unveils the very essence of electromagnetic induction. By breaking down these intricate concepts, students are provided with a comprehensive understanding of how a changing magnetic field gives rise to an electromotive force (EMF), a phenomenon at the core of electromagnetic induction. Delving further, one encounters the profound implications of Lenz's law, which dictates the conservation of energy in electromagnetic processes, offering valuable insights into the direction of induced currents and the behavior of magnetic fields. If you need help with your electromagnetic theory assignment, understanding these foundational concepts can provide a solid framework for approaching your assignments with confidence.
The exploration extends to the critical role played by magnetic fields, elucidating how variations in magnetic flux contribute to the generation of electrical currents. Beyond theoretical underpinnings, the practical applications of electromagnetic induction are unveiled, ranging from power generation to the transformative functions of transformers in electricity distribution. The connection between electromagnetic induction and the broader electromagnetic spectrum is examined, highlighting its relevance to the transmission of various waves, from radio frequencies to microwaves.
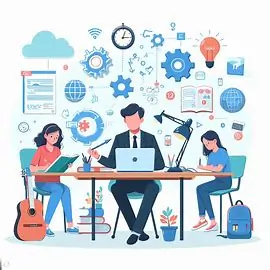
As the journey continues, a bridge is built between the theoretical and the practical, incorporating the mathematical dimensions of electromagnetic induction. Equations and formulas are dissected and explained, providing students with the tools to grasp the quantitative aspects of this captivating field. The narrative unfolds into a discussion of transformers, exploring how electromagnetic induction is harnessed to efficiently manipulate voltage levels for widespread power distribution.
Zooming out to a broader perspective, the impact of electromagnetic induction on modern technology becomes apparent, with its fingerprints evident in wireless charging, electromagnetic sensors, and other innovative applications. Yet, the journey isn't confined to technological landscapes alone; it extends into the realm of education, offering insights into effective teaching and learning strategies tailored to demystify the intricacies of electromagnetic induction. In this comprehensive exploration, the goal is clear: to make electromagnetic induction an accessible and engaging subject, simplifying complex theories for students and fostering a profound appreciation for the wonders of physics.
The Basics of Electromagnetic Induction
Discover the fundamental principles that govern electromagnetic induction, including Faraday's law and Lenz's law. We break down the key concepts to make them accessible for students at all levels. Electromagnetic induction, a fundamental concept in physics, unveils a captivating interplay between magnetic fields and electrical currents. At its core are two pivotal laws: Faraday's law and Lenz's law. Faraday's law states that a changing magnetic field induces an electromotive force (EMF) in a conductor, giving rise to an electric current. This principle forms the foundation of electromagnetic induction, linking the dynamic world of magnetism with the generation of electrical power.
Complementary to Faraday's law, Lenz's law enforces the conservation of energy in electromagnetic processes. It stipulates that the induced EMF creates a current whose magnetic field opposes the original change in magnetic flux. This inherent conservation mechanism adds depth to our understanding, revealing the intricate balance at play in electromagnetic induction.
Magnetic fields play a crucial role in this phenomenon, acting as the catalyst for inducing electrical currents. As the magnetic flux through a conductor changes, it triggers the generation of an EMF and subsequently an electric current. This dynamic relationship forms the backbone of electromagnetic induction and serves as a cornerstone for numerous technological applications.
Understanding the basics of electromagnetic induction paves the way for exploring its myriad applications, from the operation of transformers to the principles behind electric generators. This foundational knowledge not only forms an integral part of physics education but also plays a vital role in shaping the technologies that power our modern world. As we delve deeper into the intricacies of this phenomenon, we unlock the door to a realm where magnetic and electric forces harmonize, providing us with a profound insight into the workings of the natural world
Faraday's Law: Unraveling the Core Concept
Dive deep into Faraday's law of electromagnetic induction. Understand how a changing magnetic field induces an electromotive force (EMF) and the significance of this phenomenon in electrical systems. Faraday's Law of Electromagnetic Induction stands as a cornerstone in understanding the fascinating relationship between magnetic fields and electrical currents. At its essence, this law elucidates how a changing magnetic field induces an electromotive force (EMF) in a conductor, giving rise to an electric current. Coined by the renowned physicist Michael Faraday in the 19th century, this foundational principle has profound implications for electricity generation and the functioning of numerous devices.
The core concept of Faraday's Law revolves around the dynamic nature of magnetic fields. When a magnetic field surrounding a conductor undergoes change—either by movement of the conductor within the field or alterations in the field itself—an EMF is induced in the conductor. This induced EMF, measured in volts, creates an electric current if the conductor forms a closed loop. Essentially, Faraday's Law demonstrates the interconnectedness of magnetic flux changes and the generation of electrical power.
Unraveling this core concept further reveals the quantitative relationship encapsulated in the equation EMF = -dΦ/dt, where EMF represents the induced electromotive force, Φ denotes the magnetic flux, and dt signifies the change in time. The negative sign in the equation signifies Lenz's law, an integral component of Faraday's insights, indicating that the induced current opposes the change in magnetic flux that produced it.
Practical applications of Faraday's Law abound in our daily lives, from the operation of generators and alternators to technologies like magnetic card readers. Understanding this core concept not only deepens our appreciation for the elegance of electromagnetic phenomena but also empowers us to harness these principles for technological advancements that drive progress. Faraday's Law remains a testament to the profound interplay between magnetism and electricity, serving as a guiding light for innovators and scientists alike
Lenz's Law: The Conservation of Energy in Electromagnetic Processes
Explore Lenz's law and its role in electromagnetic induction. Learn how it enforces the conservation of energy, providing essential insights into the direction of induced currents and the behavior of magnetic fields. Lenz's Law, a pivotal principle within the realm of electromagnetic induction, plays a crucial role in understanding the conservation of energy in dynamic electromagnetic processes. Named after the Baltic German physicist Heinrich Lenz, this law provides profound insights into the direction of induced currents and the associated magnetic fields.
At its core, Lenz's Law states that the direction of the induced electromotive force (EMF) and the resulting current in a conductor will always oppose the change in magnetic flux that produced it. In essence, Lenz's Law enforces the conservation of energy in electromagnetic processes by introducing a self-limiting mechanism. This means that any change in the magnetic field, whether caused by motion of the conductor or alterations in the external magnetic field, results in an induced current that works against the change.
Unraveling the core concept of Lenz's Law reveals its significance in practical scenarios. Consider a coil of wire within a changing magnetic field; Lenz's Law ensures that the induced current creates a magnetic field opposing the original change in flux. This inherent opposition serves as a crucial aspect in various applications, such as electric generators and transformers, where efficient energy transfer and conservation are paramount.
The intuitive nature of Lenz's Law can be expressed mathematically in the equation EMF = -dΦ/dt. Here, EMF represents the induced electromotive force, Φ denotes the magnetic flux, and dt signifies the change in time. The negative sign in the equation emphasizes the opposing nature of the induced current, aligning with Lenz's insight into the conservation of energy.
Lenz's Law not only enhances our understanding of the interconnectedness between magnetic fields and induced currents but also underscores the elegance of nature's mechanisms to preserve energy. As we navigate the intricacies of electromagnetic processes, Lenz's Law stands as a guiding principle, highlighting the inherent balance within the dynamic world of electromagnetism.
Magnetic Fields and Their Influence on Electromagnetic Induction
Gain a comprehensive understanding of magnetic fields and their crucial role in the induction process. Explore how variations in magnetic flux contribute to the generation of electrical currents. The profound connection between magnetic fields and electromagnetic induction unveils a captivating interplay that forms the essence of various electrical phenomena. Magnetic fields play a pivotal role in influencing the generation of electric currents through the process of electromagnetic induction, providing a foundation for numerous technological applications.
Fundamentally, when a conductor is exposed to a changing magnetic field, it experiences an induced electromotive force (EMF), giving rise to an electric current. The strength and direction of this induced current are intricately linked to the characteristics of the magnetic field, creating a dynamic relationship between the two.
The influence of magnetic fields on electromagnetic induction becomes apparent when considering the concept of magnetic flux. Magnetic flux represents the quantity of magnetic field lines passing through a given surface area. As the magnetic flux through a conductor changes, whether due to movement of the conductor or alterations in the external magnetic field, it triggers the generation of an EMF. This phenomenon, described by Faraday's Law of Electromagnetic Induction, establishes the foundational link between magnetic fields and the generation of electrical power.
Exploring this relationship further, the role of magnetic fields becomes evident in shaping the direction of the induced current. This is where Lenz's Law comes into play, stating that the induced current will always work to oppose the change in magnetic flux that produced it. The inherent opposition dictated by Lenz's Law ensures a dynamic equilibrium within the system, contributing to the conservation of energy in electromagnetic processes.
The practical applications of magnetic fields in electromagnetic induction are widespread. Devices such as electric generators, transformers, and inductors rely on controlled variations in magnetic fields to induce currents and facilitate the efficient transfer of electrical energy. Additionally, magnetic fields are fundamental to the functioning of electromagnetic sensors and various technologies that utilize the principles of induction.
In conclusion, the influence of magnetic fields on electromagnetic induction is a fundamental aspect of our understanding of electromagnetism. As we delve into the intricacies of this relationship, we uncover the key role magnetic fields play in shaping the dynamics of induced currents, providing a foundation for innovations that drive technological advancements in power generation, transmission, and beyond.
Practical Applications of Electromagnetic Induction
Delve into the real-world applications of electromagnetic induction. From power generation to transformers, discover how this phenomenon powers a variety of devices and technologies we use every day.
The principles of electromagnetic induction, established by Faraday and further elucidated by subsequent physicists, have paved the way for a plethora of practical applications that shape our modern world. From powering homes to enabling advanced technologies, electromagnetic induction plays a pivotal role in numerous aspects of our daily lives.
- Electric Generators: Perhaps one of the most well-known applications, electric generators harness electromagnetic induction to convert mechanical energy into electrical energy. Rotating coils within a magnetic field induce an electromotive force (EMF), generating the electricity that powers homes, industries, and various electronic devices.
- Transformers: Transformers rely on electromagnetic induction to efficiently manipulate voltage levels. By altering the number of turns in the coils and the strength of the magnetic field, transformers step up or step down voltage, facilitating the transmission of electrical power over long distances with minimal loss.
- Induction Cooktops: Induction cooktops use electromagnetic induction to directly heat pots and pans. An alternating current in the cooktop's coil generates a magnetic field, inducing electric currents in the metal cookware, and thereby producing heat.
- Wireless Charging: The concept of electromagnetic induction is at the heart of wireless charging technologies. Devices such as smartphones and electric toothbrushes utilize induction to transfer power without the need for physical connectors.
- Magnetic Card Readers: Magnetic card readers, commonly found in credit card machines and access control systems, rely on electromagnetic induction to read information encoded on magnetic stripes. As the card is swiped through the reader, the changing magnetic field induces an electrical current that corresponds to the encoded data.
- Electromagnetic Sensors: Various sensors, including those used in automotive applications (such as ABS brakes and proximity sensors), rely on electromagnetic induction to detect changes in magnetic fields. These changes are then translated into electrical signals for control and feedback purposes.
- Eddy Current Brakes: Eddy current brakes leverage the principles of electromagnetic induction to create resistance and slow down moving objects without physical contact. These brakes are often used in high-speed trains and roller coasters for precise and efficient speed control.
- Metal Detectors: Metal detectors utilize electromagnetic induction to identify the presence of metallic objects. When a metal object enters the detector's magnetic field, it induces eddy currents, creating a detectable signal that alerts the user to the presence of metal.
- Magnetic Resonance Imaging (MRI): In the medical field, MRI machines use powerful magnets and electromagnetic induction to generate detailed images of internal body structures. The interaction between the magnetic field and hydrogen atoms in the body produces signals that are translated into images.
- Power Harvesting: Electromagnetic induction is also employed in power harvesting systems, where it is used to convert ambient energy, such as vibrations or magnetic fields, into electrical power for low-energy electronic devices like sensors and IoT devices.
These practical applications underscore the versatility and importance of electromagnetic induction in diverse fields, showcasing its transformative impact on technology, energy, and daily conveniences.
Electromagnetic Spectrum: A Window into the World of Waves
Connect the dots between electromagnetic induction and the broader electromagnetic spectrum. Learn how induction is related to the transmission of radio waves, microwaves, and more.
The electromagnetic spectrum stands as a vast and fascinating continuum of energy, offering a comprehensive view of the various forms of electromagnetic waves that permeate the universe. As we explore this spectrum, we delve into the diverse range of electromagnetic waves, each characterized by unique properties, applications, and interactions with matter.
At one end of the spectrum are radio waves, known for their long wavelengths and applications in communication, broadcasting, and radar systems. Moving towards shorter wavelengths, microwaves come into play, serving not only in communication but also in technologies like microwave ovens, where they interact specifically with water molecules for heating.
The intermediate range encompasses infrared radiation, crucial in thermal imaging, remote sensing, and even the warmth we feel from the sun. As we progress further, visible light emerges—a tiny sliver in the spectrum but fundamental to human vision and the vibrant colors of the world around us.
Beyond visible light lie the shorter wavelengths of ultraviolet (UV) radiation, associated with effects such as sunburn and utilized in applications like sterilization. X-rays, with even shorter wavelengths, find application in medical imaging, revealing the internal structures of the human body. At the extreme end, we encounter gamma rays, produced by nuclear processes and employed in medical treatments and certain types of imaging.
The electromagnetic spectrum is not only a scientific curiosity but a powerful tool for exploration and communication. Radio astronomers use radio waves to study celestial bodies, while astronomers observe the universe across different wavelengths, providing insights into cosmic phenomena. Telecommunications rely on a broad range of frequencies within the spectrum for wireless communication, satellite transmission, and global connectivity.
Understanding the electromagnetic spectrum is integral to grasping the interconnectedness of these waves and their impact on our daily lives. It serves as a window into the invisible world of waves, revealing the rich tapestry of energy that surrounds us. Moreover, advancements in technology continually push the boundaries of our exploration, utilizing different regions of the spectrum for applications ranging from high-speed data transmission to medical diagnostics.
In essence, the electromagnetic spectrum serves as both a scientific tool and a source of inspiration, offering a profound glimpse into the dynamic and interconnected nature of electromagnetic waves that shape the fabric of our universe
Exploring the Mathematics Behind Electromagnetic Induction
Demystify the mathematical aspects of electromagnetic induction. We break down the equations and formulas, making it easier for students to grasp the quantitative aspects of this fascinating field. The fascinating world of electromagnetic induction is not only characterized by its conceptual elegance but also by the mathematical precision that underlies its principles. Delving into the mathematical expressions associated with electromagnetic induction enriches our understanding, providing a quantitative framework to describe the dynamic interplay of magnetic fields and electrical currents.
At the heart of the mathematical representation of electromagnetic induction is Faraday's Law, a cornerstone principle established by Michael Faraday. The law articulates the relationship between the induced electromotive force (EMF) and the rate of change of magnetic flux through a conductor. Mathematically, this relationship is expressed as:
EMF = −dΦ/dt/
Here, EMF represents the induced electromotive force, Φ denotes the magnetic flux, and dt represents the change in time. The negative sign in the equation signifies Lenz's Law, elucidating that the induced current opposes the change in magnetic flux that produced it.
Further mathematical insights arise when considering the magnetic flux (Φ), which is the product of the magnetic field (B) and the area (A) through which the magnetic field lines pass:
Φ=B⋅A
This equation underscores the dependence of magnetic flux on both the strength of the magnetic field and the area perpendicular to the field.
For a more intricate understanding, the expression for induced EMF can be expanded for a coil with multiple turns, introducing the concept of the magnetic flux linkage (Λ):
EMF = −dΛ/dt
The magnetic flux linkage is the product of the magnetic flux and the number of turns in the coil:
Λ=N⋅Φ
In this context, N represents the number of turns in the coil.
These mathematical formulations not only provide a means to quantify electromagnetic induction but also offer a foundation for solving real-world problems in electrical engineering and physics. The equations enable the calculation of induced EMF, helping engineers design efficient electrical systems and devices based on electromagnetic induction principles.
Exploring the mathematics behind electromagnetic induction, therefore, unveils a structured and rigorous approach to understanding the quantitative aspects of this phenomenon. It empowers scientists and engineers to harness the principles of electromagnetic induction in a precise and controlled manner, driving innovations in power generation, transmission, and the development of transformative technologies.
Transformers: Powering the World with Electromagnetic Induction
Uncover the role of transformers in electricity distribution. Understand how electromagnetic induction is harnessed to step up or step down voltage levels, ensuring efficient power transmission. In the realm of electrical engineering, transformers stand as silent but indispensable giants, leveraging the principles of electromagnetic induction to revolutionize the transmission and distribution of electrical power. These devices, which come in various sizes and capacities, play a pivotal role in ensuring efficient and reliable electricity supply on a global scale.
At the heart of a transformer lies the elegant interplay between magnetic fields and electrical currents, a phenomenon elucidated by Faraday's Law of Electromagnetic Induction. Transformers consist of two coils—primary and secondary—intimately linked by a magnetic core. When an alternating current (AC) flows through the primary coil, it produces a changing magnetic field. This dynamic magnetic field induces an electromotive force (EMF) in the secondary coil, giving rise to a corresponding AC in the secondary circuit. The beauty of this process lies in the conservation of energy, a principle upheld by Lenz's Law, ensuring the harmony and efficiency of the transformation.
Transformers serve a dual purpose in the world of electricity. Firstly, they facilitate the stepping up or stepping down of voltage levels, allowing for the efficient transmission of electrical power over long distances. High-voltage transmission reduces energy loss, as power is inversely proportional to the square of the voltage according to the power equation P = VI. Subsequently, at local distribution points, transformers step down the voltage to safer and usable levels for homes, businesses, and industries.
The significance of transformers extends beyond their role in voltage manipulation. They contribute to grid stability by balancing loads and voltages, enhancing the overall reliability of power systems. Large power transformers, often found in substations, are critical components in the electricity infrastructure, ensuring that the energy generated at power plants reaches end-users with minimal losses.
In addition to their central role in power distribution, transformers have diverse applications in various electronic devices and industries. They power electronic appliances, provide isolation between different components of electronic circuits, and drive innovations in technology. Their adaptability and efficiency make them integral to the functioning of a myriad of electronic systems.
As we marvel at the seamless operation of our electronic devices and the reliability of our power grids, it's worth acknowledging the silent contribution of transformers. These devices, grounded in the elegant principles of electromagnetic induction, form the backbone of our electrified world, powering our homes, industries, and technological advancements. In essence, transformers exemplify the fusion of science and engineering, demonstrating how fundamental principles can be harnessed to shape the modern landscape of electricity
Electromagnetic Induction in Education: Teaching and Learning Strategies
Discover effective teaching and learning strategies for electromagnetic induction. Whether you're a student seeking a simplified approach or an educator looking for engaging methods, we've got you covered.
Embark on a journey through the world of electromagnetic induction, where complex theories are made accessible and captivating for students of all levels.
As educators seek to convey the intricacies of electromagnetic induction to students, employing effective teaching and learning strategies becomes paramount. This fascinating yet complex topic in physics requires an approach that combines theoretical understanding with hands-on experiences, fostering both engagement and comprehension. Here are several strategies to enhance the teaching and learning of electromagnetic induction:
- Hands-on Demonstrations: Conducting hands-on experiments and demonstrations is a powerful way to illustrate the principles of electromagnetic induction. Simple setups, such as using a coil and magnet to generate a current, can bring abstract concepts to life and enhance students' grasp of the underlying principles.
- Interactive Simulations: Incorporate interactive simulations and virtual experiments into the curriculum. Online tools and simulations allow students to manipulate variables, observe outcomes, and visualize electromagnetic induction processes in a dynamic and engaging manner.
- Visual Aids and Multimedia: Enhance traditional lectures with visual aids, animations, and multimedia presentations. Diagrams, charts, and videos can provide a visual representation of electromagnetic phenomena, making abstract concepts more accessible to students.
- Real-World Applications: Connect theoretical concepts to real-world applications. Discuss the practical uses of electromagnetic induction in technologies like transformers, generators, and wireless charging. Highlighting the relevance of the subject matter can motivate students and deepen their understanding.
- Collaborative Learning: Foster collaborative learning environments where students can work together to solve problems, conduct experiments, and discuss concepts related to electromagnetic induction. Group activities and discussions can provide diverse perspectives and enhance the overall learning experience.
- Problem-Solving Exercises: Integrate problem-solving exercises and challenges into the curriculum. Presenting students with real-world problems that require the application of electromagnetic induction principles encourages critical thinking and reinforces their problem-solving skills.
- Practical Applications Project: Assign a project that involves designing a practical application based on electromagnetic induction. This could be anything from a simple electric generator model to a more complex system. Encouraging creativity and innovation allows students to apply theoretical knowledge in a meaningful way.
- Guest Speakers and Industry Insights: Invite guest speakers from relevant industries to share their insights and experiences related to electromagnetic induction. Real-world perspectives can inspire students and provide a glimpse into potential career paths in physics and engineering.
- Interactive Workshops and Labs: Organize interactive workshops and laboratory sessions where students can actively engage with electromagnetic induction phenomena. Providing opportunities for hands-on exploration reinforces theoretical concepts and encourages a deeper understanding.
- Technology Integration: Embrace technology by using simulations, virtual reality, and educational apps that focus on electromagnetic induction. Integrating technology not only caters to the preferences of modern learners but also enhances the overall learning experience.
By combining these teaching and learning strategies, educators can create a dynamic and inclusive learning environment that empowers students to grasp the principles of electromagnetic induction with enthusiasm and confidence.
Conclusion
In conclusion, the exploration of electromagnetic induction unveils a captivating realm where the interplay of magnetic fields and electrical currents shapes the foundation of modern physics and technology. Faraday's Law and Lenz's Law, governing this phenomenon, provide a theoretical framework that not only enriches our understanding of fundamental principles but also powers a multitude of practical applications.
From the silent giants, transformers, stepping up and stepping down voltages to ensure efficient power transmission, to the subtle yet essential electromagnetic sensors detecting changes in magnetic fields, the impact of electromagnetic induction is pervasive in our daily lives. It extends beyond practical applications to the intricate mathematics that quantifies and describes the dynamic relationships between magnetic flux, electromotive force, and time.
As we journey through the electromagnetic spectrum, we discover a diverse tapestry of waves, each with its unique properties and applications. From radio waves connecting us through communication to X-rays unraveling the mysteries of internal structures, the spectrum serves as a window into the invisible world of electromagnetic phenomena.
Transformers, powered by electromagnetic induction, emerge as the unsung heroes of electricity distribution, silently ensuring the reliable flow of power to our homes and industries. Their adaptability and efficiency underscore the synergy between science and engineering, showcasing how fundamental principles can be harnessed to shape the modern landscape.
In the realm of education, effective teaching and learning strategies elevate the comprehension of electromagnetic induction. From hands-on experiments and collaborative learning to interactive simulations and real-world applications, these strategies aim to inspire students and provide a holistic understanding of this captivating subject.
In essence, electromagnetic induction, with its blend of theoretical elegance and practical significance, stands as a testament to the beauty and utility of physics. As we continue to unravel its complexities and integrate its principles into technological advancements, electromagnetic induction remains a driving force, powering the world and illuminating the path to future innovations.