Unlocking the Potential of Atomic Physics: Exploring Real-World Applications and Case Studies
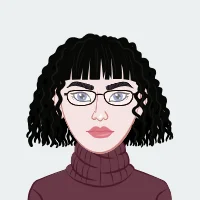
Unlocking the potential of atomic physics heralds a journey into the intricate fabric of the universe, where the fundamental principles governing the behavior of atoms reveal a wealth of possibilities for real-world applications. This exploration delves into the heart of innovation, showcasing how atomic physics serves as the bedrock for technological marvels that shape our modern world. From the microscopic precision of nanotechnology to the macroscopic effects influencing our daily lives, the impact of atomic physics is far-reaching. The medical field harnesses the power of atomic physics to heal and advance treatments, while sustainable energy solutions draw inspiration from atomic principles for cleaner and more efficient power generation. Case studies in areas such as quantum computing, space exploration, and atomic clocks illustrate the diverse ways in which atomic physics is at the forefront of cutting-edge developments. As we navigate this intricate realm, we uncover the incredible potential and challenges that lie ahead, providing a glimpse into the future frontiers of atomic physics research and its transformative influence on our technological landscape. If you need help with your atomic physics assignment, understanding the diverse applications and implications of atomic physics is essential for tackling assignments effectively and appreciating the profound impact of atomic principles on various technological advancements.
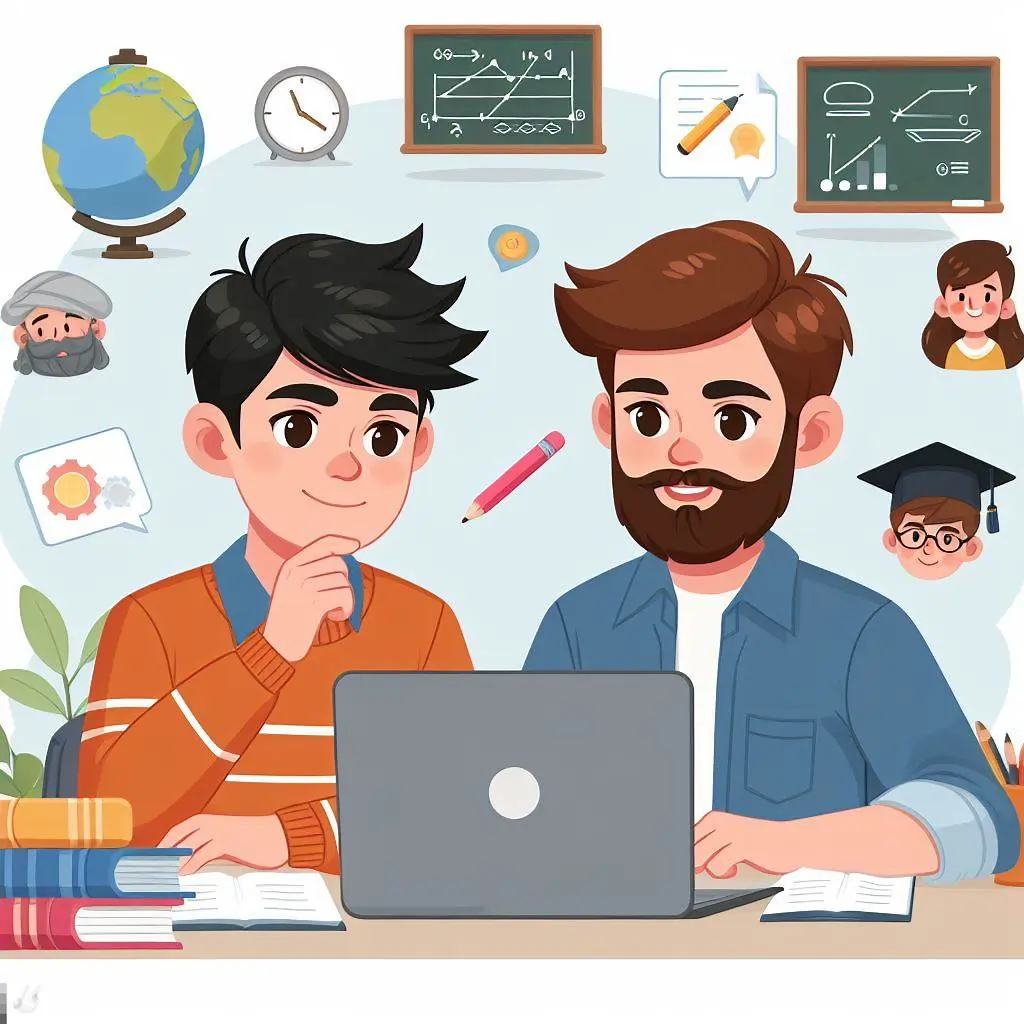
Moreover, the precision afforded by atomic physics is exemplified in the realm of nanotechnology, where scientists manipulate individual atoms to create materials and devices with unparalleled accuracy and efficiency. This microscopic precision has far-reaching implications, influencing industries ranging from electronics to medicine. The development of atomic clocks, utilizing the predictable vibrations of atoms, has revolutionized timekeeping, ensuring accuracy at the quantum level and impacting everything from global communication networks to satellite navigation systems.
In the ever-expanding field of space exploration, atomic physics plays a pivotal role. Case studies in this domain illuminate how atomic principles contribute to the design of spacecraft, communication systems, and even propulsion methods. Beyond our planet, atomic physics provides essential tools for understanding the composition of celestial bodies, unraveling the mysteries of the cosmos.
The advent of quantum computing represents another frontier where atomic physics is unlocking unprecedented computational power. Harnessing the unique properties of atomic particles, quantum computers have the potential to solve complex problems at speeds unimaginable with classical computing. This promises transformative breakthroughs in fields such as cryptography, optimization, and artificial intelligence.
As we delve into the various facets of atomic physics in action, it becomes evident that its applications are not confined to a single discipline but permeate virtually every aspect of our modern world. Yet, with these advancements come challenges, both ethical and scientific, that demand careful consideration. Navigating this dynamic landscape requires collaboration among scientists, engineers, and policymakers to ensure the responsible and sustainable development of atomic technologies. Thus, exploring the real-world applications and case studies of atomic physics opens not only a door to incredible possibilities but also invites a thoughtful dialogue about the responsible stewardship of this powerful knowledge in shaping the future.
The Fundamentals of Atomic Physics
The fundamentals of atomic physics provide a cornerstone for understanding the intricate nature of matter at its most fundamental level. At its core, atomic physics explores the behavior of atoms, the building blocks of all matter in the universe. This field delves into the principles that govern the structure, properties, and interactions of atoms, unraveling the mysteries of the subatomic world. The exploration begins with a focus on the atom's constituent particles, such as protons, neutrons, and electrons, and how their arrangement influences the characteristics of different elements. Understanding atomic structure is crucial for comprehending the diverse phenomena observed in nature and engineered systems.
Quantum mechanics, a fundamental theory in atomic physics, provides a framework to describe the behavior of particles at the atomic and subatomic scales. Concepts like wave-particle duality, superposition, and entanglement challenge classical intuitions, opening new avenues for understanding the peculiar nature of the quantum realm. Moreover, atomic physics plays a vital role in elucidating the principles of spectroscopy, revealing how atoms interact with electromagnetic radiation and enabling scientists to probe the composition of distant stars, galaxies, and other celestial bodies.
The fundamentals of atomic physics lay the groundwork for a myriad of applications across various scientific and technological domains. From the development of cutting-edge technologies to advancements in medical diagnostics and treatments, a solid understanding of atomic physics is indispensable. As we explore the intricacies of atomic behavior, we unlock not only the secrets of the subatomic world but also the potential for groundbreaking innovations that continue to shape our understanding of the universe and improve the quality of life on Earth.
Technological Marvels: Atomic Physics at the Heart of Innovation
Technological marvels stand as a testament to the profound influence of atomic physics at the heart of innovation. At the core of this exploration lies the transformative power of understanding and manipulating the behavior of atoms. In the realm of technology, atomic physics serves as the guiding force behind a myriad of breakthroughs that shape our modern world.
One of the most compelling applications is found in the field of electronics. The miniaturization of electronic components, essential for the development of powerful and compact devices, relies heavily on the principles of atomic physics. Semiconductor materials, which form the basis of modern electronics, leverage the controlled manipulation of atoms to achieve specific electronic properties. This, in turn, has fueled the rapid evolution of computers, smartphones, and a multitude of other gadgets that have become integral to our daily lives.
Furthermore, atomic physics plays a pivotal role in materials science. The ability to engineer materials at the atomic level has led to the creation of innovative substances with tailored properties, such as superconductors and advanced alloys. These materials find applications in diverse industries, from aerospace to healthcare, paving the way for advancements in manufacturing, transportation, and medical technologies.
The advent of nanotechnology exemplifies how atomic physics is pushing the boundaries of innovation. Scientists and engineers are now capable of manipulating individual atoms to design nanoscale structures with unprecedented precision. This capability not only opens the door to new materials and devices but also holds immense potential for addressing challenges in medicine, environmental science, and beyond.
As we continue to unlock the potential of atomic physics, technological marvels continue to emerge, revolutionizing the way we live, work, and communicate. From quantum computing to the development of advanced sensors and imaging technologies, atomic physics remains at the forefront of the technological frontier, driving innovation and shaping the future of our increasingly interconnected world
Healing with Atoms: Applications of Atomic Physics in Medicine
The marriage of atomic physics and medicine represents a groundbreaking intersection where the precision and properties of atoms are harnessed to advance diagnostics, treatments, and overall healthcare. In this realm, atomic physics plays a pivotal role in revolutionizing medical technologies and therapies, offering unprecedented insights into the human body at the atomic and molecular levels.
One of the most notable applications is found in medical imaging. Techniques such as Magnetic Resonance Imaging (MRI) rely on the principles of atomic physics to generate detailed images of internal structures within the body. By exploiting the magnetic properties of atomic nuclei, particularly hydrogen atoms, MRI provides non-invasive, high-resolution images critical for diagnosing a myriad of medical conditions, from neurological disorders to musculoskeletal injuries.
In the realm of nuclear medicine, atomic physics takes center stage in diagnostic and therapeutic procedures. Positron Emission Tomography (PET) scans, for instance, involve the detection of gamma rays emitted by positron-emitting isotopes introduced into the body. This not only allows for the visualization of metabolic processes but also aids in the early detection of diseases such as cancer. Similarly, targeted radiotherapy utilizes the precise delivery of radiation to specific areas within the body, leveraging the properties of radioactive isotopes to destroy cancerous cells while sparing surrounding healthy tissues.
The development of nanomedicine, an emerging field that involves the use of nanoscale materials for medical applications, is deeply rooted in atomic physics. Nanoparticles engineered at the atomic level can be designed to interact selectively with specific cells or tissues, enabling targeted drug delivery and enhancing the efficacy of therapeutic interventions.
Beyond diagnostics and treatment, atomic physics also contributes to the understanding of biological processes at the molecular level, unraveling the complexities of diseases and facilitating the development of novel pharmaceuticals. In essence, healing with atoms in medicine signifies a paradigm shift, where the precision and versatility of atomic physics are harnessed to enhance the accuracy of diagnoses, improve treatment outcomes, and pave the way for innovative medical solutions that hold the promise of a healthier future
Powering the Future: Atomic Physics in Energy Generation
The quest for sustainable and efficient energy sources has propelled atomic physics into the forefront of innovations that hold the key to powering the future. Atomic physics plays a pivotal role in understanding and harnessing the incredible energy stored within the nucleus of atoms, offering solutions that address the growing global demand for clean and reliable energy.
At the heart of this exploration is nuclear energy, where the process of nuclear fission releases vast amounts of energy by splitting the nucleus of heavy atoms. Nuclear power plants utilize this principle to generate electricity on a large scale. The controlled fission reactions produce heat, which, in turn, is used to produce steam, driving turbines that generate electrical power. The efficiency and energy density of nuclear fission make it a compelling option for providing baseload power without the greenhouse gas emissions associated with traditional fossil fuels.
Moreover, ongoing research in atomic physics is paving the way for advancements in nuclear fusion, often considered the holy grail of clean energy. Fusion reactions, akin to those occurring in the sun, hold the promise of virtually limitless and environmentally friendly energy. Scientists are working on developing fusion reactors that can mimic the conditions necessary for sustained fusion reactions, offering a potential game-changer for the global energy landscape.
Beyond nuclear technologies, atomic physics contributes to the development of advanced materials for energy storage and conversion. For instance, atomic-scale engineering is employed in the design of batteries, fuel cells, and solar cells, enhancing their performance, efficiency, and sustainability. The understanding of atomic interactions also plays a role in the exploration of novel materials for energy capture, storage, and transmission, pushing the boundaries of what is possible in the realm of renewable energy.
As we stand on the precipice of a changing energy paradigm, the role of atomic physics in energy generation underscores not only the technological advancements but also the imperative of transitioning towards cleaner and more sustainable solutions. By unlocking the potential of atomic energy, we embark on a journey towards a future where power is not only abundant but also environmentally responsible, laying the foundation for a more resilient and sustainable world
Beyond the Microscope: Macroscopic Effects of Atomic Physics
While atomic physics is often associated with the microscopic world of atoms and subatomic particles, its influence extends far beyond the confines of the microscope, exerting profound effects on the macroscopic scale. This exploration into the macroscopic effects of atomic physics unveils a spectrum of phenomena and applications that shape the physical properties of matter, influence diverse fields, and impact our daily lives.
At the forefront of these macroscopic effects is the concept of phase transitions. Atomic interactions and arrangements determine how substances transition between solid, liquid, and gas phases, influencing the behavior and properties of materials. Understanding these transitions is crucial in fields ranging from materials science to meteorology, where changes in phase dictate weather patterns and atmospheric conditions.
Furthermore, the principles of atomic physics govern the thermal properties of matter. The study of heat transfer and thermodynamics, rooted in atomic interactions, allows us to engineer systems for efficient energy transfer and design technologies ranging from refrigeration to aerospace propulsion.
In the macroscopic realm, atomic physics plays a pivotal role in the development of new materials with tailored properties. By manipulating the arrangement of atoms, scientists and engineers can create materials with specific mechanical, electrical, and magnetic characteristics, influencing the design and functionality of everything from structural materials to electronic devices.
Atomic physics also contributes to the understanding of fluid dynamics, where the behavior of liquids and gases on a large scale is intricately linked to the interactions of individual atoms. This knowledge finds applications in fields as diverse as aeronautics, environmental science, and civil engineering.
Moreover, the study of macroscopic effects in atomic physics extends to the exploration of quantum mechanics at larger scales. Quantum coherence and entanglement, once considered phenomena confined to the microscopic world, are now being explored for potential applications in quantum computing, communication, and sensing on a macroscopic scale.
In essence, the macroscopic effects of atomic physics underscore the interconnectedness of the micro and macro worlds. As we unravel the mysteries of atomic interactions, we gain insights that transcend the microscopic scale, impacting the design of materials, technologies, and systems that shape the macroscopic world around us. This interconnected understanding forms the basis for innovations that continue to redefine our understanding of the physical universe and drive advancements across a multitude of scientific and technological disciplines
Atomic Precision: Case Studies in Nanotechnology
Nanotechnology, a field characterized by manipulating matter at the nanoscale with precision down to individual atoms, represents a frontier where atomic physics unfolds its transformative potential. This exploration into atomic precision through case studies in nanotechnology reveals how scientists and engineers are leveraging the unique properties of atoms to create materials, devices, and applications with unprecedented precision and functionality.
One remarkable case study lies in the development of nanoscale materials with tailored properties. By precisely arranging atoms, researchers can engineer materials with characteristics not found in bulk matter. Quantum dots, for example, exhibit quantum mechanical properties due to their size and composition, making them valuable in applications ranging from advanced imaging technologies to solar cells. The precise control over the atomic structure enables the customization of materials for specific purposes, opening new avenues in material science and engineering.
Another fascinating application is found in nanoelectronics, where the miniaturization of electronic components reaches atomic dimensions. Transistors, the building blocks of electronic devices, are now being designed at the atomic scale, leading to faster, more energy-efficient, and smaller electronic devices. Case studies in this realm showcase the intricate interplay between atomic physics and information technology, paving the way for the continued evolution of computing.
In the field of medicine, nanotechnology offers groundbreaking solutions through case studies such as targeted drug delivery. Nanoparticles, engineered with precision at the atomic level, can carry medications directly to specific cells or tissues, reducing side effects and increasing therapeutic efficacy. This level of precision holds promise for personalized medicine and the treatment of diseases at the molecular level.
Furthermore, nanotechnology is making strides in energy applications. Case studies reveal innovations in nanomaterials for energy storage, such as high-capacity batteries and supercapacitors, as well as advancements in nanoscale devices for efficient energy conversion. These applications highlight the potential of atomic precision in addressing global energy challenges.
As we delve into these case studies, it becomes evident that atomic precision in nanotechnology is not merely a theoretical concept but a tangible and powerful tool driving innovation across diverse disciplines. The ability to manipulate matter at the atomic scale opens up possibilities that were once unimaginable, paving the way for a future where technologies are not only smaller and more efficient but also tailored to meet the specific needs of various industries and societal challenges.
Atomic Clocks and Timekeeping: Precision on a Quantum Scale
The relentless pursuit of precision in timekeeping has led to a remarkable convergence of atomic physics and horology, giving rise to the era of atomic clocks. This exploration into atomic clocks and timekeeping delves into the fascinating intersection of quantum mechanics and the measurement of time, revealing how these ultra-precise instruments have become the bedrock of modern time standards.
At the heart of atomic clocks is the concept of atomic resonance. These timekeeping marvels rely on the vibrations of atoms, typically cesium or rubidium, which resonate at specific and incredibly stable frequencies when exposed to electromagnetic radiation. The quantum nature of these vibrations allows atomic clocks to achieve an unparalleled level of accuracy, with deviations on the order of one second in millions of years.
One of the most iconic examples of atomic timekeeping is the cesium atomic clock. By exposing cesium atoms to microwave radiation, scientists can precisely measure the resonance frequency associated with the electron transitions within the cesium atoms. The resulting oscillations serve as the basis for defining the length of a second, forming the foundation for international timekeeping standards.
The evolution of atomic clocks has led to even more precise timekeeping devices, such as optical lattice clocks. These instruments use laser light to trap and cool atoms, achieving precision at the quantum scale. Optical lattice clocks, based on transitions in optical frequencies, offer accuracies that surpass traditional atomic clocks, opening new frontiers in fundamental physics and scientific research.
The application of atomic clocks extends far beyond accurate timekeeping. Global navigation systems, such as the Global Positioning System (GPS), rely on the synchronization of atomic clocks aboard satellites to provide precise location data on Earth. Additionally, advancements in telecommunications, scientific research, and space exploration owe much of their success to the unparalleled precision afforded by atomic clocks.
As we peer into the intricate world of atomic clocks, it becomes evident that their precision on a quantum scale has redefined our perception and measurement of time. The marriage of atomic physics and timekeeping not only ensures the synchronization of our daily lives but also fuels scientific discoveries and technological innovations that rely on the utmost precision in the measurement of time. In essence, atomic clocks stand as a testament to humanity's quest for precision, pushing the boundaries of our understanding of the quantum world and reshaping the landscape of modern technology.
Atomic Physics in Space Exploration: Case Studies from the Cosmos
The vastness of space serves as an awe-inspiring canvas for the application of atomic physics, where the fundamental principles governing the behavior of atoms play a pivotal role in unraveling the mysteries of the cosmos. This exploration into atomic physics in space exploration reveals compelling case studies that showcase how atomic interactions and processes are integral to our understanding of the universe and the technologies that propel us beyond Earth.
One notable case study involves the analysis of starlight through the discipline of spectroscopy. By breaking down the light emitted by stars into its component colors, scientists can identify the unique spectral lines produced by different atomic elements. This process, rooted in atomic physics, enables astronomers to determine the composition, temperature, and motion of celestial objects. Spectroscopy has been instrumental in studying the atmospheres of distant planets, the life cycles of stars, and the dynamics of galaxies, providing crucial insights into the vast cosmic tapestry.
Atomic physics also plays a crucial role in propulsion systems for spacecraft. Ion propulsion, for example, relies on the principles of atomic physics to generate thrust. By ionizing atoms and propelling them through electromagnetic fields, spacecraft equipped with ion drives achieve greater speeds and fuel efficiency compared to traditional chemical rockets. Case studies in this realm showcase the transformative impact of atomic physics on space exploration, enabling longer missions, faster travel, and the exploration of distant reaches of our solar system.
Furthermore, the study of cosmic rays, energetic particles originating from outside our solar system, involves a deep understanding of atomic interactions. Cosmic rays, consisting of protons, electrons, and atomic nuclei, provide valuable information about distant astrophysical phenomena. Detectors aboard spacecraft and satellites measure the energy and types of particles, offering insights into the composition and conditions of the interstellar medium and distant celestial bodies.
Atomic physics also contributes to our exploration of planets and moons within our solar system. Instruments on robotic spacecraft, such as X-ray and gamma-ray spectrometers, leverage atomic interactions to analyze the elemental composition of planetary surfaces. These measurements help scientists unravel the geological history of planets, identify potential resources, and understand the conditions that shaped their landscapes.
As we continue to venture into the cosmos, the case studies of atomic physics in space exploration underscore its indispensable role in expanding our understanding of the universe. From unraveling the secrets of distant galaxies to enabling the propulsion systems that drive our spacecraft, atomic physics stands as an essential tool in our quest to explore the cosmos and unlock the mysteries that lie beyond our celestial doorstep.
Quantum Computing: Harnessing Atomic Physics for Unprecedented Power
In the realm of computing, a revolutionary frontier is unfolding with the advent of quantum computing, a paradigm that relies on the principles of atomic physics to unlock computing power previously deemed unattainable. This exploration into quantum computing delves into how harnessing atomic physics brings forth unprecedented computational capabilities, promising a transformative shift in our approach to solving complex problems.
At the core of quantum computing is the quantum bit or qubit, the fundamental unit of information in quantum systems. Unlike classical bits, which can exist in a state of 0 or 1, qubits can exist in a superposition of both states simultaneously, thanks to the principles of superposition in quantum mechanics. This unique characteristic exponentially increases the computing capacity of quantum systems, enabling them to process vast amounts of information in parallel.
Another key concept is entanglement, a phenomenon where qubits become interconnected and the state of one qubit instantaneously influences the state of another, regardless of the distance between them. This interconnectedness allows quantum computers to perform complex calculations and solve problems at speeds that classical computers would find impractical.
Quantum computing case studies showcase its potential to revolutionize fields such as cryptography, optimization, and artificial intelligence. Shor's algorithm, for instance, threatens current encryption methods by efficiently factoring large numbers, a task that would take classical computers an impractical amount of time. Quantum computers also excel at solving optimization problems, making them invaluable for tasks such as route planning, resource allocation, and financial modeling.
Moreover, quantum machine learning, a burgeoning field at the intersection of quantum computing and artificial intelligence, leverages the unique capabilities of quantum systems to process and analyze vast datasets more efficiently than classical counterparts. This holds promise for advancements in drug discovery, pattern recognition, and other data-intensive applications.
The development of practical quantum computers is a complex challenge, requiring precise control over the behavior of quantum bits and mitigation of errors caused by environmental factors. However, ongoing research and advancements in the field of atomic physics, including techniques such as trapped ions and superconducting circuits, are propelling us closer to realizing the full potential of quantum computing.
As we navigate the uncharted territory of quantum computing, the marriage of atomic physics and information processing promises not only unprecedented computational power but also a paradigm shift in our ability to address complex problems that were once deemed insurmountable. Quantum computing stands as a testament to the limitless possibilities that emerge when we harness the intricate dance of atoms for the betterment of scientific discovery and technological innovation.
Conclusion
In conclusion, the exploration of atomic physics in various realms, from medicine to space exploration and quantum computing, unveils a tapestry of remarkable advancements and transformative applications. The fundamental principles governing the behavior of atoms serve as the foundation for groundbreaking innovations that have reshaped our understanding of the universe and propelled us into the forefront of technological frontiers.
From the precision of atomic clocks, defining the very essence of time, to the microscopic precision of nanotechnology, manipulating matter at the atomic scale, atomic physics plays a central role in shaping our modern world. In medicine, the marriage of atomic precision and healthcare has given rise to breakthroughs in diagnostics, treatments, and targeted drug delivery, offering new avenues for personalized medicine.
The macroscopic effects of atomic physics, influencing phase transitions, thermal properties, and material science, underscore the interconnectedness of the micro and macro worlds. Space exploration, fueled by atomic physics, unveils the cosmic mysteries through spectroscopy, propulsion systems, and the study of celestial bodies, showcasing the indispensable role of atomic principles in our quest to understand the cosmos.
The emergence of quantum computing as a paradigm shift highlights the power of harnessing atomic physics for unprecedented computational capabilities. Quantum bits, entanglement, and superposition redefine the possibilities of information processing, opening doors to advancements in cryptography, optimization, and artificial intelligence.
As we stand on the precipice of a future shaped by atomic physics, challenges and ethical considerations accompany these advancements. Responsible stewardship of this powerful knowledge is imperative to ensure the sustainable and ethical development of technologies that leverage atomic principles.
In essence, the journey through atomic physics in action reveals a landscape of endless possibilities, where the manipulation and understanding of atoms lead to innovations that transcend traditional boundaries. Whether healing with atoms in medicine, powering the future through atomic energy, or unraveling the mysteries of the cosmos, atomic physics serves as a guiding light, illuminating the path towards a future where precision, innovation, and transformative breakthroughs continue to redefine the possibilities of human achievement.